by Shane L. Larson
It has been just more than a week since we told the world about our great discovery. It was a cold winter morning in Washington DC, the temperature hovering just below freezing. In a room at the National Press Club, the world press had gathered, and at the behest of NSF Director, Frances Córdova, LIGO Executive Director, Dave Reitze, took to the podium.
“Ladies and gentlemen. We have detected gravitational waves. We did it!” Mic drop. (Well, he should have; in the movie dramatization, he will. You can watch the moment here on YouTube, or the full press conference.)
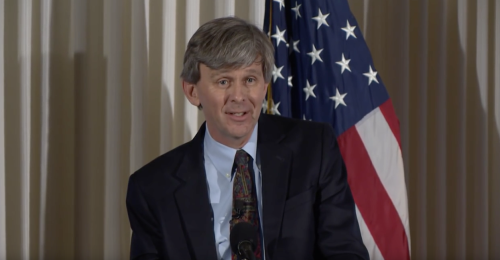
Dave Reitze makes the announcement to the world that LIGO had detected gravitational waves. “We did it!”
So began a ninety minute press conference delivering the news of the first gravitational wave detection to the world. In the days that followed, social media and press outlets exploded in a veritable tidal wave of excitement and awestruck wonder. On twitter, the hashtags #gravitationalwaves, #LIGO, and #EinsteinWasRight have accumulated more than 70 million tweets in just one week.
Everyone has the same sense that we scientists have — this is a doorway, now open, to a Universe we have only imagined. Beyond the threshold are certainly things we have predicted and speculated about, but also many wonders yet to be found or understood.
We have done our best to explain what we are doing with LIGO, and how it works. We have made a Herculean effort to describe the astrophysical significance of the discovery. We have tried mightily to explain what Einstein’s ideas about spacetime and gravity are all about.
But this is hard stuff to think about, it is hard stuff to understand, and it is hard stuff to explain. It is well outside our normal everyday experience, so it is easy to feel like your brain is melting.
You shouldn’t worry that these things are hard to understand. It took physicists 41 years to even decide gravitational waves were real, and then another 59 years to build an experiment capable of detecting them. There is no doubt these are hard, brain melting matters. But the beauty of the discovery of gravitational waves is that this can be understood!
A large number of my colleagues in LIGO (and myself) have spent the last week collecting and responding to questions emailed to us, asked in public forums, and delivered on social media (if you have more questions, ask in the comments below, or please email question@ligo.org). All of them are thoughtful, genuine, and demonstrate a pleasing curiosity and wonder about the nature and workings of the Cosmos. I am constantly amazed by the questions people ask.
Here are a few of the more common brain-melters we have been asked, and some meager attempt to answer them. The questions are marked in red, to make them easy to find. Some responses are more complicated than others, and you may or may not want to read them all. They are here to help stem the meltdown, if you find your brain is still reeling. 🙂
What does this mean for ordinary folks? Far and away, this is the most common question I’ve been asked, particularly from the press. What does this mean for the world? How will this help my golf game?
LIGO’s discovery is what we call “fundamental physics.” It is a discovery that tells us something about how the Universe works and why it behaves the way it does. Figuring out how to use knowledge like that to make your life better or turning it into a gadget that’s useful in your kitchen or garage takes time — we’ve only just now made the first detection of gravitational waves, and are trying to wrap our brains around it. Scientists and engineers will have to think a long time, maybe decades, before they can make this knowledge “useful for everyday life.” That’s always how it works with scientific discoveries. How it will impact our everyday lives is not for us to know — that is for the future.
That is not to say that there isn’t some amazing future application. We only have to look at the history of general relativity itself to know the truth of this. Einstein worked out general relativity between 1905 and 1915. This was an age before cars and electricity were mainstays in everyday life. Yet Einstein had the where-with-all to understand that gravity could be thought of as the warpage of spacetime, and that one consequence of that warpage is clocks tick at different speeds depending on how strong the gravity is.
Did you know that little, obscure fact of general relativity is used by you and most other people every, single day? It is an essential part of how the GPS in your phone works. It took nearly a hundred years for the “fundamental physics” we call general relativity to be turned into an essential piece of technology that now gets millions of us from place to place in the world every day. Without GPS and general relativity, you’d still be navigating using paper maps. Einstein had to rely on his neighbor to tell him where to find a pub; you have a smartphone.
Is it really that important? I think this is one of the most important discoveries in astronomy in the last 100 years. It is as important as discovering that there are other galaxies beyond the Milky Way, it is as important as discovering the expansion of the Universe, it is as important as discovering the Cosmic Microwave Background.
The reason I think this is just about everything you’ve ever heard about the Universe, or seen a picture of, has been discovered using LIGHT. Telescopes are just instruments that do what your eyes do (collect light), though telescopes collect much more light than your eye or collect light that your eye cannot see (like infrared or ultraviolet light).
Gravitational waves are different — none of us have a “gravitational wave detector” as part of our bodies. Gravitational waves are something that we predicted should exist, and we built an experiment that showed us our ideas were right. The beginning of gravitational wave observations will change how we see the Universe in ways that we cannot yet imagine.
As a scientist and a teacher, I can appreciate the importance and utility of the collection of knowledge. But LIGO’s discovery goes far beyond the mere acquisition of yet another fact to post on Wikipedia. What the scientists and engineers working on LIGO have done was often regarded as impossible to do. But as Dr. Córdova intoned at the LIGO press conference, we took a big risk. Through a judicious application of sweat, brains, and stubbornness, we endured a decades long effort to design a machine to do the impossible. We encountered countless challenges and obstacles, and diligently overcame every single one of them to arrive at this day. That should make every person sit up a little bit straighter and prouder. That should make every single person aware that whatever challenges or problems we face on our small world, we have the means to overcome them, if we have the will to commit our time and brains and resources to them.
The black hole collision LIGO observed was more than 50 times brighter than all the stars in the Universe. How can that be? The comparison is “the gravitational energy released by the merger is about 50 times the energy released by all the stars in the Universe during the same time.” This is an example of a “Fermi problem” which astrophysicists use all the time to figure out if our numbers are right when we are doing complex calculations.
![The night sky over the Pando Forest in central Utah. Pando is an 80,000 year old aspen grove -- it has seen almost 30 million nights like this one, but very little has changed. The constellations change over thousands of years, but the sky is still full of stars, and the Milky Way still arches over the sky, giving the impression that the Universe is unchanging. [Image: Shane L. Larson]](https://writescience.files.wordpress.com/2015/03/pandoforest.jpg?w=254&h=381)
The night sky over the Pando Forest in central Utah. Pando is an 80,000 year old aspen grove — it has seen almost 30 million nights like this one, bathed in the light of the stars of the Milky Way. [Image: Shane L. Larson]
If you express the luminosity of the black holes (3 solar masses in just about 20 milliseconds) as a “wattage,” the brightness is about 3.6 x 1049 watts, or about 1023 times brighter than the Sun.

The Hubble Extreme Deep Field (XDF). We can use images like this to estimate the total number of stars in the Cosmos.
Now suppose we make the assumption that all the stars in the Universe are just like the Sun. This isn’t true, of course — some are brighter, some are dimmer, but on the average this is a good starting guess. There are about 100 billion stars in a galaxy like the Milky Way, and if you look at an image like the Hubble Extreme Deep Field, there are on order 100 billion galaxies in the Universe. So there are 100 billion x 100 billion = 1022 stars in the Universe. If each one of them is the brightness of the Sun, the total brightness of stars in the Universe is 1022 times the brightness of the Sun.
But we said the black hole merger seen by LIGO was 1023 times brighter than the Sun, so: 1023/1022 = 10. The black hole merger was 10x brighter than all the stars in the Cosmos. With a careful calculation, we could get the 50 number you hear from LIGO, but 10 is pretty close. This is the nature of Fermi problems — they don’t give you the exact number, but they quickly get you close to the exact number so you can understand the Universe.
What do you mean “spacetime is stretching LIGO’s arms?” What is spacetime? Spacetime is the substrate, the matrix upon which everything in the Universe is built — as we like to say, spacetime is the “fabric of the Cosmos.” It is, of course, easy to say that, but difficult to wrap your brain around. We’re used to not thinking about space at all; it is the nothing between everything. But it is exactly that nothing of which we speak — if we were not here, if nothing were here, there is still space.

Imagine a gravitational wave shooting through LIGO, directly out of the screen at you. (A) When there are no waves, the arms are at their fixed lengths. (B) When the wave first hits LIGO, the spacetime in one arm stretches and in the other arm compresses. This changes how long it takes light to go from the corner to the end of the arms and back again. (C) As the wave passes by, the arms change back and forth between stretching and compressing.
How do you measure the length of something in space? Most of the time we use a ruler or a tape measure. You lay it down along the thing you are interested in, like LIGO’s arms, and you see how many it takes. Imagine that you put down kilometer markers along LIGOs arms, just like you see on the highway — one at 0km, 1km, 2km, 3km and 4km. When spacetime between the ends of LIGO changes, the entire arm stretches. You still think the arm is 4 kilometers long, because the markers are still evenly spaced (the spacing is just larger than it was before, though you may not be aware of it). We need a way to measure the stretching without relying on the kilometer markers.
![Visualization of LIGO interferometry. (A) When no gravitational wave is present, the laser timing is set up to make a "dark fringe" at the output [square panel on the right]. (B) At the output, the light is like waves canceling each other out. (C) When a wave stretches or compresses the arms, it changes how the light is added together at the output. [Frames from video by Caltech/LIGO]](https://writescience.files.wordpress.com/2016/02/ligo_interferometerframes22.jpg?w=500&h=835)
Visualization of LIGO interferometry. (A) When no gravitational wave is present, the laser timing is set up to make a “dark fringe” at the output [square panel on the right]. (B) At the output, the light is like waves canceling each other out. (C) When a wave stretches or compresses the arms, it changes how the light is added together at the output. [Frames from video by Caltech/LIGO]

A simple demonstration of how sensitive interference can be to small shifts in space. These interference patterns are made with overlapping patterns of regular circles (as opposed to moving waves) and create Moiré patterns. Here the horizontal dark region in the left image is analogous to LIGO’s “dark fringe.” The difference between the left and right image is a shift of only 0.05 inches, but the pattern difference is easy to see. What was a “dark fringe” now has a sliver of white, indicating the shift happened. [Image: S. Larson]
They said the stretching that LIGO measured was a fraction of the width of a proton. But I remember from Chemistry that atoms are always moving, so how can you make such a precise measurement? Remember that LIGO is not measuring the distance shift in single atoms — it is watching the mirror, which is comprised of many atoms, each of which is moving exactly as you remember from Chemistry.

Everyone on a boat is doing their own thing, but they are all moving together as the boat moves on the waves.
When we make our measurements, we are looking at the behaviour of many, many photons that have travelled down the arm together, hit the mirrors, and made the return journey. Sure — some of the atoms are going one way, and some are going some other way, but overall they are all moving together, going wherever gravity is pushing the center of mass of the mirror. When we read out the light, we are looking at all of those photons that hit the mirror at the same time and using that information to determine where the mirror is.
It’s a bit like having a big gravitational wave discovery party on a boat. If you are on the shore, watching all the physicists and engineers having a good time, you see they are all going every which way on the deck. But they are all on the boat, which moves them all together in response to the underlying waves of the sea.
Will this help with time travel? quantum gravity? Einstein’s great discovery with general relativity was the idea that gravity can be described as the interaction of mass with the shape and warpage of spacetime. The unification of space and time into a single entity — spacetime — is a huge conceptual leap that is sometimes hard to come to grips with because of the way we think about space and time.
In our everyday lives we measure space with rulers and car odometers, and we measure time with wristwatches and calendars. If they are the same thing, why don’t we measure them the same way? The idea that the two are connected takes some getting used to, though as I like to remind people: when you go somewhere, you are usually comfortable saying your destination is “25 minutes” away or saying “20 miles” away!

You think about travel as travelling through space or travelling through time without even noticing! You are used to being a “spacetime traveller.”
Since gravitational waves are moving ripples, propagating warpage in spacetime, it is natural to ask: can this discovery can help us understand space and time? Can we understand “time travel” and “warp drive?”
Time itself, despite being part of general relativity, is still a great mystery to us. But what we often forget is that we are time travelers. Even as you are reading this, you are traveling through time from this moment, heading toward next Tuesday. It is not possible, so far as we know, to go backward toward last Friday, and that is a great mystery. It appears to be true based on experimental evidence, but we don’t yet understand how the laws of Nature — general relativity — tell us that. So in as much as gravitational waves will dramatically improve our understanding of how spacetime works and behaves, that deeper understanding could lead us down a path of thinking that will ultimately give us more insight into the mystery of time.
In a similar way, the LIGO detection does not address the enduring questions about the microscopic, quantum nature of gravity. The gravitational waves are a “big world” phenomenon, created by strongly gravitating astrophysical objects. But based on our experience with other quantum physics, we expect that there will be a clear (though not now obvious) connection between quantum gravity and general relativity. The more we expand our understanding of general relativity, it becomes more likely we will stumble on the deep connections that would lead to ultimately understanding quantum gravity.
—————————————
I encourage you to continue asking your favorite physicist your questions and share what you learn. Also, send questions to: question@ligo.org
Remember: no question is a dumb question. If you are wondering something, I’ll bet you a jelly donut someone else has the exact same question!
Poor analogy, but here goes:
Is there a concept of spacetime getting thinner or thicker as it is stretched or compressed by gravity? If the gravitational waves make one arm ‘longer’, is there now just more spacetime between the mirrors, does it get stretched and thinner like an elastic? Does spacetime itself exhibit different properties under the influence of gravitational waves?
I had not understood that it was solely about the distance traveled and timing of the photons. I had thought it also had to do with a tiny change in position, caused by the gravitational waves, whose impact was then seen when the photons hooked up to create the interference pattern, not just due to timing, but to a slight change in direction as well.
Thanks for the great post and keeping your work so accessible.
–TAPP
We often think of spacetime in the “fabric” analogy, because it is easy to visualize and see physical effects in simple experiments that are analogous to what happens in full 4D spacetime, but there are parts of the analogy that are not entirely price. The fact that any stretchy fabric you might use to visualize this has a thickness is one of those weaknesses. We we talk about spacetime like a stretchy piece of fabric, we are in reality completely constraining our attention and discussion to the surface of the fabric; the thickness does not play a role.
But your question and your intuition is good — the behaviour of the fabric has something to do with its properties that reflect how it is made. Spacetime, like fabric, has a tension when it stretches; we like to say “spacetime is stiff” meaning it is hard to stretch (that’s why it takes big masses to make things happen).
Spacetime’s properties when gravitational waves are present are different because it has a shape it did not have before; the fact that we can measure the gravitational waves is a reflection of this fact. By timing how long it takes the laser light to fly up and down the arms, we are measuring that the spacetime has changed — it has stretched, curved, from its original shape.
Thanks for visiting the blog!
Pingback: Allgemeines Live-Blog vom 18.-26. Februar 2016 | Skyweek Zwei Punkt Null
Pingback: The Cosmic Classroom on Boxing Day | Write Science